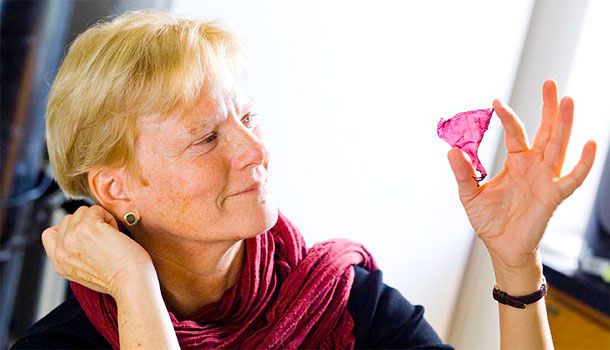
Alison Butler
Chemistry and Biochemistry
Santa Barbara
Alison Butler couldn’t stop playing with the magnets and iron filings in preschool, and she’s still fascinated by iron today. Most living things, both on land and at sea, need iron to grow and thrive. And Butler, a UC Santa Barbara professor of chemistry and biochemistry, has spent much of her research life examining exactly how organisms go about getting it out of their environment.
“One of the best parts of doing science is when you get an answer that’s totally unexpected, and that’s really when the scientific thought process kicks in, and when you must look at things in new ways,” she says. “That’s the part of my interaction with my students that is really exciting. I feel like my group is all in it together; we don’t have hierarchy, we’re just all on this great quest to decipher the physical and biological world we live in.”
Butler grew up in a family of scientists: her father was one of the first biology faculty hired by UC San Diego. Her research, she explains, is at the interface of inorganic chemistry and biochemistry, and a lot of it revolves around “transition metals.” Chemistry students will remember these metals from the periodic table – scandium, titanium, vanadium, chromium, manganese, iron, copper, nickel, cobalt and zinc. Butler’s particularly interested in how marine bacteria use transition metals in combination with biochemical players like peptides, proteins and enzymes to extract iron from the ocean.
Question:
What attracted you to chemistry?
Answer:
I started off in immunology but soon found myself drawn to chemistry, which was very exact, mathematical, predictive and colorful. Transition metals, which I went on to research, make wonderfully colored complexes. Organic chemists are always trying to achieve the purest, whitest material; they don’t want any contamination. But for inorganic chemists, you can make really intensely colorful compounds with transition metals, enzymes and proteins. There are blue copper proteins, and red iron proteins. The colors really caught my eye.
Question:
What led you to the ocean, and from seaweed to bacteria?
Answer:
When I first started, I wanted to work on a new metal in biology, and the first vanadium enzyme had just been discovered: vanadium bromoperoxidase. It was found in Dutch seaweed. So I flew over to Holland one weekend, went out to a dike, picked up seaweed and brought it home — I hadn’t realized yet how widespread this enzyme was, I could have found it off the coast of California.
Question:
Why do bacteria need metals?
Answer:
All bacteria are thought to require iron for growth. Bacteria devote a tremendous amount of metabolic energy to get iron. Bacteria growing in aerobic environments like the ocean are presented with an extreme challenge of how to get iron, because iron is scarce in the surface ocean (and not very soluble) and is predominantly present as iron oxides. Think of a tiny micron scale bacterium trying to absorb something as large as a rust particle. So the bacteria produce chelating ligands called siderophores, to pull iron out of particulate matter.
Question:
Paint a picture of a siderophore for us. What does it look like? How does it work?
Answer:
The ones we’ve found in our marine bacteria often have a head and a tail. The head group is water soluble, and hangs out in the ocean to get iron. Think of your hand hanging out in the water, trying to claw an iron atom. The head is the iron chelating component, so it binds with the iron atom, and now you have a fist. We don’t know much about the function of the tail, but we do know it’s a fatty acid tail, making this siderophore amphiphilic, or both water- and fat-loving. The fatty greasy part is what can embed in the membrane of the bacteria. The bacteria also produce a receptor protein channel which recognizes the siderophore when it comes time to deliver the iron.
Question:
Can throwing a bunch of iron in the ocean save us from climate change?
Answer:
According to the “iron hypothesis,” if you added iron to the oceans it would stimulate photosynthetic growth, consume carbon dioxide and possibly contribute to limiting the greenhouse effect. There is no doubt that iron is a very important component in regulating the global carbon cycle; however, too little is known about the possible negative consequences or long-term effects of seeding the oceans with iron, to recommend this. We still need to study what limits or promotes carbon cycling, and clearly iron is involved at some level.
Question:
Does your research have any terrestrial or human health applications?
Answer:
One of the very first responses of many organisms, including humans, to a bacterial infection is for the host to sop up all its iron. The host tries to limit the availability of iron to an invading pathogen. So that’s a bacterium having to grow in a very low-iron environment. This is similar to the ocean, also a very low-iron environment.
So it’s interesting that another kind of bacteria, apart from marine bacteria, that make amphiphilic siderophores are certain pathogens such as mycobacteria that cause tuberculosis or acinetobacteria, such as those prevalent in hospital situations. Building on what we’ve learned from marine bacteria, we might be able to disrupt the synthesis of siderophores in these bacteria.
Question:
What chemistry question keeps you up at night?
Answer:
As we described in a recent issue of Nature, we discovered that siderophores with alpha- [or a-] hydroxy carboxylic acids are photoreactive when bound to iron. In other words, they can’t stand up to light. They break down. So they’re not only more vulnerable to structural change in ultraviolet light, but also metabolically expensive molecules to make, from a biosynthetic point of view.
So why would bacteria make molecules that have this photoreactive vulnerability when bound to the metal they’re expressed to get, if they aren’t using the photoreactivity somehow? This is a question that keeps me up at night. I mean, bacteria can’t afford to make molecules that get destroyed if they aren’t using the process to their advantage in some way. Maybe it promotes iron uptake through another pathway. We don’t really know yet, but I’m hoping to find out.